Green and fast biodiesel production at room temperature using soybean and Jatropha curcas L. oils catalyzed by potassium ferrate
Green and fast biodiesel production at room temperature using soybean and Jatropha curcas L. oils catalyzed by potassium ferrate
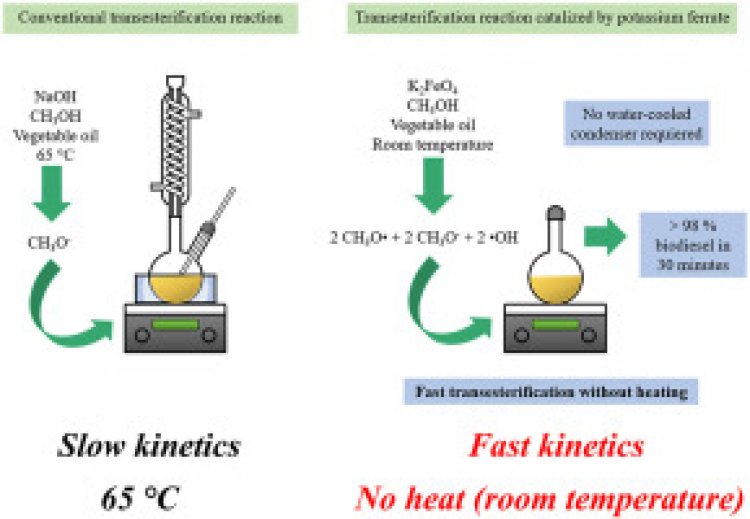
Gutiérrez-López, A. N., Mena-Cervantes, V. Y., González-Espinosa, M. A., Sosa-Rodríguez, F. S., Vazquez-Arenas, J., Rodríguez-Ramírez, R., & Hernández-Altamirano, R. (2022). Green and fast biodiesel production at room temperature using soybean and Jatropha curcas L. oils catalyzed by potassium ferrate. Journal of Cleaner Production, 372, 133739. https://doi.org/10.1016/j.jclepro.2022.133739
Graphical abstract
Introduction
Economic development and population growth have led to a rapid increase in energy demand, which is mostly obtained from petroleum-derived fuels. The combustion of fossil fuels significantly contributes to the emission of greenhouse gases, causing environmental impacts such as global warming and climate change, whereby it is necessary to diversify the energy matrix by incorporating alternative sources of energy (Chozhavendhan et al., 2020). In this direction, biodiesel is a carbon neutral and biodegradable fuel obtained from renewable sources that can be used pure or mixed with fossil diesel (Singh et al., 2018). Its implementation can improve air quality as a consequence of reducing emissions of sulfur oxides, particulate matter, aromatics, carbon monoxide, carbon dioxide, and unburned hydrocarbons (Rouhany and Montgomery, 2019).
Biodiesel is comprised of a mixture of fatty acid alkyl esters produced by transesterification of vegetable oils or animal fats with methanol subjected to acid, basic or enzymatic catalysis (Gebremariam and Marchetti, 2018). Currently, biodiesel is produced using homogeneous basic catalysts such as sodium hydroxide due to its high commercial availability and affordable cost, however, it undesirably reacts with free fatty acids to produce soaps (sodium carboxylates), thus, requiring additional purification operations by removing impurities with water (Tacias-Pascacio et al., 2019). Recent efforts are focused on making biodiesel production more environmentally friendly, preferentially selecting heterogeneous catalysts to avoid the drawbacks of homogeneous base catalysis, including null catalyst recovery and reuse for various reaction cycles (Changmai et al., 2020). Heterogeneous catalysts are typically made up of alkaline earth metal oxides such as CaO (Kouzu et al., 2017), MgO (Rasouli and Esmaeili, 2019), and SrO (Roschat et al., 2018); transition metal oxides including TiO2 (Carlucci et al., 2019), ZnO–SiO2 (Justine et al., 2021), and Na2ZnSiO4 (Rodríguez-Ramírez et al., 2020); hydrotalcite (Navajas et al., 2018); mixed metal oxides containing Sr–Ce (Banerjee et al., 2019), Sr–Ti (Sahani et al., 2020), and Ca–Mg (Sudsakorn et al., 2017); and biomass-based catalysts consisting of banana trunk ash (Rajkumari and Rokhum, 2020) and eggshell (Yaşar, 2019), among others. However, these materials still present some disadvantages, such as deactivation due to moisture and free fatty acids (FFA), slow reaction rate associated with diffusion limitations, high alcohol to oil molar ratio, complex synthesis and fabrication, and leaching of active catalyst sites (Changmai et al., 2020). And particularly, they are used at around 65 °C to promote the formation of a methoxide ion attacking the triglyceride to form the biofuel, which is responsible of most of the energy consumption of the process.
Potassium ferrate (K2FeO4) is a strong oxidizing agent widely used in environmental applications such as water and waste-water treatment because it does not form any harmful by-products, provides efficient inactivation of microorganisms, degrades and oxidizes organic and inorganic impurities, and removes suspended colloidal particles via coagulation-flocculation process (Jiang et al., 2006). Also, potassium ferrate has attracted attention in organic synthesis. The oxidation of alcohols to form aldehydes or ketones by potassium ferrate has been likewise reported (Bartzatt et al., 1985; Delaude et al., 1995). Recently, methanol oxidation routes have been theoretically described, in which methoxide radical (CH3O•) can be produced as an intermediate before formaldehyde formation (Ohta et al., 2001). The methoxide radical could lead to the production of methyl esters in a considerable shorter time even at room temperature due to its high reactivity compared to the methoxide ion (CH3−).
At present, soybean, palm oil, and rapeseed oil are the dominant biodiesel feedstocks because of their high availability in many geographical areas (Joshi et al., 2017). Jatropha curcas is another promising inedible energy crop since it displays low irrigation requirements and can be grown on land unsuitable for growing food. Also, it is widely distributed in Mexico and Central America, Asia, and Africa, and its seeds contain approximately 40–50% good quality oil (Ewunie et al., 2021). The economic competitiveness of biodiesel against fossil diesel is one of the determining factors promoting a sustainable energy transition using renewable fuels. The price of this biofuel is determined by feedstock, unit operations integrating the production process, production volume, food prices, government incentives, as well as research and technological development costs, etc. (Rouhany and Montgomery, 2019). Accordingly, it is of paramount importance to reduce the heating consumption in the transesterification reaction producing biodiesel, in order to favorably impact costs that benefit its profitability and applicability. This has stemmed the development of these processes at room temperature, although, there is a lack of information regarding this issue. To this concern, Piker et al. (2016) obtained a conversion of 98 wt% and 97 wt% using SBO and waste cooking oil (WCO) respectively (1:6 oil: methanol molar ratio), and eggshells as catalyst (5.8% w/w) after 11 h at room temperature (25 ± 3 °C). The eggshell was utilized during 10 and 5 cycles for SBO and WCO. Laskar et al. (2018) reported 98 wt% conversion of SBO into methyl esters (1:6 oil to methanol molar ratio) at room temperature (28 °C) using waste snail shell as catalyst (3 wt%) after 7 h. The catalyst was recycled 8 times. Recently, Laskar et al. (2020) obtained 98 wt% conversion of SBO (1:6 oil to methanol molar ratio) at 28 °C using mango peel ash (6 wt%) after 4 h and the catalyst was used for 4 cycles. Although low-cost catalysts are used and heating is not required in the aforementioned studies, the reaction time is still long, which implies high energy consumption due to stirring.
Therefore, the current study reports the pseudo-catalytic performance of K2FeO4 at room temperature using refined SBO and crude JCO for biodiesel production, with the aim of using a sustainable pseudo-catalyst without heating in a short reaction time. A Box-Behnken experimental design was employed to achieve the maximum conversion through the interaction analysis of the following variables: stirring rate (125–700 RPM), K2FeO4 dosage (0.15–6 wt%), and methanol to oil molar ratio (6:1 to 16:1). Once obtained the optimized reaction conditions, conversion was measured at 0, 1, 5, 10, 15, 30, 45, 60, and 90 min. Additionally, physicochemical characterization of each oil and its respective methyl esters was carried out. Likewise, the K2FeO4 is characterized using XRD and SEM analysis.
Section snippets
Determination of physicochemical properties of soybean oil and Jatropha curcas L
The refined SBO from the Nutrioli brand was purchased from a local market, while the raw JCO was obtained as mentioned below: The seeds were collected in Zacatepec, Morelos, Mexico. Then, they were cracked to carefully separate the kernels and shells. The crushed kernels were placed in a round-bottom flask with ethanol in proportion 1:5 w/v. The mixture was heated at 60 °C under magnetic stirring at 700 RPM for 6 h, and then, it was allowed to cool down to room temperature before being vacuum
Determination of physicochemical properties of soybean oil and Jatropha curcas L
The fatty acid composition of vegetable oils determines their physicochemical properties. Several studies have reported that palmitic (C16), oleic (C18:1) and linoleic acid (C18:2) are the major components in SBO and JCO, which fluctuate depending on environmental conditions (Ovando-Medina et al., 2013). The physicochemical properties of both oils, SBO and crude JCO are summarized in Table 1. The acid number is used to determine the free fatty acids (FFA) content in the oil, and it is expressed
Conclusions
Potassium ferrate has been successfully shown to catalyze the transesterification reaction at room temperature of SBO and JCO. Maximum conversion under optimized reaction conditions of 98.35% and 98.17% were obtained in 1 h, respectively. However, kinetic experiments revealed that conversion of 98.31% is reached without significant variation over time using SBO after 5 min. On the other hand, a conversion of 92.63% was obtained at 5 min for JCO, reaching its maximum conversion (98.65%) at
CRediT authorship contribution statement
Adriana N. Gutiérrez-López: Investigation, Funding acquisition, Experimental data acquisition, Data treatment, Data curation. Violeta Y. Mena-Cervantes: Conceptualization, Formal analysis, Collection and Discussion of XRD and SEM analyses. Mario A. González-Espinosa: Determination of physicochemical parameters of biodiesel. Fabiola S. Sosa-Rodríguez: Formal analysis, Collection and Discussion of Chromatography analysis. Jorge Vazquez-Arenas: Methodology, Writing – original draft, preparation,
Declaration of competing interest
The authors declare that they have no known competing financial interests or personal relationships that could have appeared to influence the work reported in this paper.
Acknowledgments
The authors thank the Consejo Nacional de Ciencia y Tecnología de México for the financial support through the Project No. 315787.
References (49)
- et al.
Effect of biodiesel production parameters on viscosity and yield of methyl esters: jatropha curcas, Elaeis guineensis and Cocos nucifera
Alex. Eng. J.
(2015) - et al.
Process dynamic investigations and emission analyses of biodiesel produced using Sr–Ce mixed metal oxide heterogeneous catalyst
J. Environ. Manag.
(2019) - et al.
Flash points of mixtures containing ethyl esters or ethylic biodiesel and ethanol
Fuel
(2012) - et al.
A review on influencing parameters of biodiesel production and purification processes
Current Research in Green and Sustainable Chemistry
(2020) - et al.
Oxidation of organic substrates with potassium ferrate (VI) in the presence of the K10 montmorillonite
Tetrahedron Lett.
(1995) - et al.
Factors affecting the potential of Jatropha curcas for sustainable biodiesel production: a critical review
Renew. Sustain. Energy Rev.
(2021) - et al.
Online monitoring of transesterification reaction by medium-resolution benchtop 1H NMR and NIR spectroscopy
Fuel Process. Technol.
(2020) - et al.
Economics of biodiesel production: Review
Energy Convers. Manag.
(2018) - et al.
The influence of straight vegetable oil fatty acid composition on compression ignition combustion and emissions
Fuel
(2015) - et al.
The exploration of potassium ferrate(VI) as a disinfectant/coagulant in water and wastewater treatment
Chemosphere
(2006)
Cited by (1)
-
Exploiting the Waste Biomass of Durian Shell as a Heterogeneous Catalyst for Biodiesel Production at Room Temperature
2023, International Journal of Environmental Research and Public Health
Recommended articles (6)
-
Research article
Mesoscale impact of the sea surface on the performance of offshore wind farms
Journal of Cleaner Production, Volume 372, 2022, Article 133741 -
Research article
Lithium production, electricity consumption, and greenhouse gas emissions: An imperious role of economic globalization
Journal of Cleaner Production, Volume 372, 2022, Article 133689 -
Research article
Assessment framework and empirical analysis of the ecological protection importance for key town agglomerations in China
Journal of Cleaner Production, Volume 372, 2022, Article 133682 -
Research article
Modeling and optimization of electrodeionization process for the energy-saving of ultrapure water production
Journal of Cleaner Production, Volume 372, 2022, Article 133754 -
Research article
Removal of concentrated Cr(III) from real tannery wastewater using abiotic and anaerobic processes with native microbial consortia
Journal of Environmental Chemical Engineering, Volume 9, Issue 1, 2021, Article 104626 -
Research article
Efficient and economic transesterification of waste cooking soybean oil to biodiesel catalyzed by outer surface of ZSM-22 supported different Mo catalyst
Biomass and Bioenergy, Volume 167, 2022, Article 106646